The labs pointing to a greener future for Africa
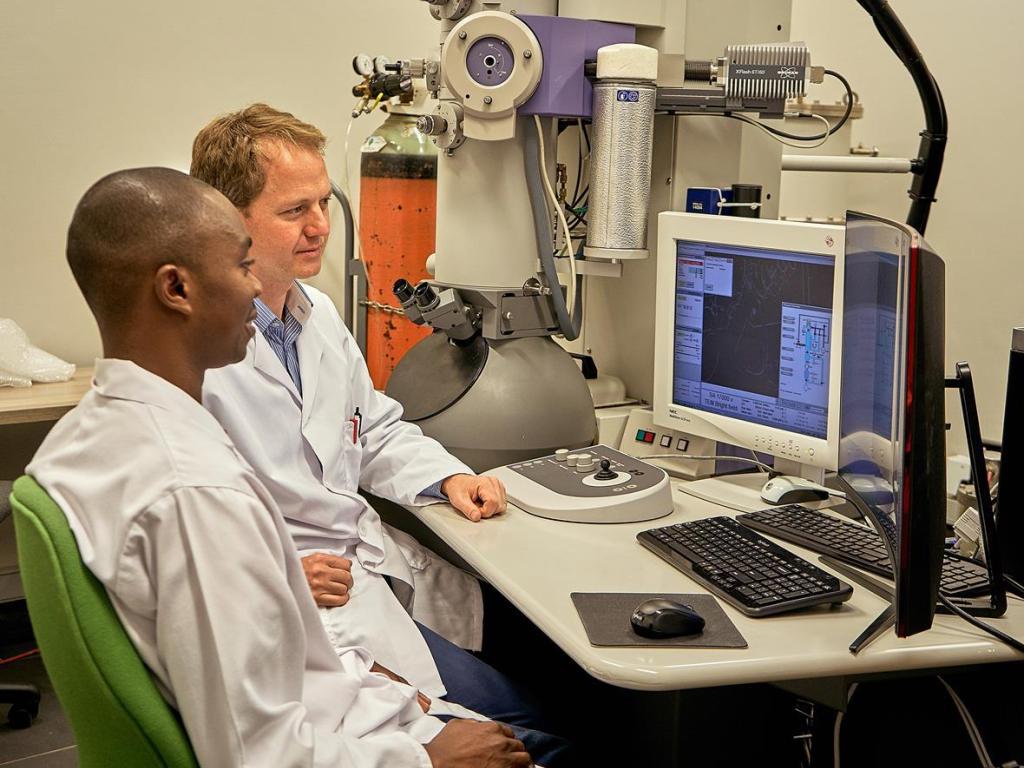
While working on plant nitrilases in the laboratory of Markus Piotrowski at the Ruhr-University Bochum in Germany 10 years ago, Woodward observed that the substrate specificity could be modified, not only by modifying amino acids near the active site, but also by altering amino acids in a ‘hot spot’ far from the active site. This latter alteration changed the helical pitch of the filament.
Even before working as a research fellow, Mulelu had been fascinated by the structure of helical nitrilases during his final year as an undergraduate, especially those that detoxified cyanide, which have potential to clean up pollution from mining and electroplating. During his doctoral studies he worked on a bacterial cyanide hydratase enzyme, a member of the nitrilase superfamily known to specifically speed up the conversion of cyanide into formic acid and ammonia. Mulelu determined the structure at near atomic resolution (3.15Å) by cryoEM together with Julian Reitz at the Goethe University Frankfurt in Germany. A significant difference between the plant and bacterial nitrilases was an insertion of only five amino acidsin an interfacial surface of the helical subunit near the ‘hot spot’ identified by Woodward.
Woodward and Kirykowicz decided to explore this systematically by targeting selected amino acids for directed evolution. This technique connects the survival of bacteria expressing nitrilase variants to the successful conversion of substrates and thus enables a detailed analysis of the correlation between substrate specificity and amino acid variation at specific locations.
Mulelu and Woodward asked the question: ‘How did this work? How did these translate into differences that could account for the ability of these enzymes to distinguish between substrates that differ by only one carbon atom?’
The answer lay in determining the structure by cryoEM. The GCRF Start grant enabled Mulelu and Woodward to travel to the electron bio-imaging centre at the UK’s Diamond Light Source with their precious samples and return with terabytes of image data. The result was a three-dimensional map of stunning clarity which was easily interpreted. ‘We saw the enzyme at high enough resolution to build in the coordinates of the individual atoms in the protein,’ Mulelu says.
The results show how the insertion creates a flap over the active site of an adjacent subunit in the helix enabling one of the ‘hot spot’ side chains to penetrate the heart of the active site of the neighbouring subunit. Thus the specificity pocket is dependent on the interaction of two adjacent subunits in the helical assembly and enabling the specificity to be tuned by modifying amino acids that are far removed in sequence space from the catalytic site.
These results set the tone for new research possibilities. Woodward had his sights on ‘designer’ nitrilases’ to find biotechnology solutions which could lead to sustainable changes in the lives and livelihoods of Africans. A major challenge in protein engineering is the generation of enzymes with novel substrate specificities. A detailed knowledge of several nitrilase fibres will enable in silico generation of nitrilase variants with tailored specificity and other properties.
Cleaning up cyanide
This approach has been adopted by Lenye Dlamini, currently doing her PhD in the structural biology research group at the University of Cape Town. She picked up Mulelu’s work and that of Michael Benedik’s group at Texas A&M University in the US who used directed evolution to increase protein stability. Her goal is to use computers to rapidly identify specific amino acid changes that will lead to increased stability of the cyanide degrading enzymes and thus will make them better agents for the control of environmental cyanide pollution.
As efforts to reduce human impact on the environment demands more sustainable and environmentally friendly waste management systems, as well as the bioremediation of damaged ecosystems, biocatalysis can be a replacement for conventional chemical synthesis in industries in line with the UN’s Sustainable Development Goals.
Dlamini says the engineering of a cyanide-degrading nitrilase enzyme can be used to reclaim cyanide waste in the textile, electroplating and gold-mining industries, which use cyanide and produce cyanide-containing waste. Spills or unsafe disposal results in environmental pollution causing damage to natural resources, ecosystems and poses a threat to human health.
The direct impact of Dlamini’s research project is environmental, but it has broader implications in a variety of industries. The demand for biological agents and processes that will replace conventional processes of managing waste and chemical synthesis is also growing. ‘Enzymes, including the nitrilases, have taken centre-stage in this regard and present not just an environmentally benign alternative but one that produces better reaction products,’ she says.
Nitrilases are highly specific in the enantiomers and regions on compounds that they bind to, leading to more specific reaction products. Using predictive biophysical methods based on structural knowledge of cyanide degrading nitrilases, Dlamini intends to measurably improve their tolerance of non-optimal temperature ranges and enhance the operational stability of the enzyme so that they can be routinely used for safe and cheap disposal of cyanide waste.